Five questions for Thomas Ayres
Stargazing takes on an entirely different meaning for Thomas Ayres, who looks beyond the beauty of the night sky, focusing instead on the “invisible” ultraviolet and X-ray light emitted by stars in our galaxy.
His early passion was fueled by his father, a science fiction buff who shared stories of alien worlds, and by an uncle, a science teacher with a love of astronomy. At a young age, Ayres wanted to become director of the “Lunar Observatory,” a facility, he admits with a touch of sadness, that still is only a dream.
Now, as a senior researcher at the Center for Astrophysics and Space Astronomy (CASA) at CU Boulder, his interests are sparked by objects trillions of miles away, with decidedly more pizzazz than our airless, lifeless moon. His studies highlight one piece of the puzzle of the sun’s “space weather,” which can affect planet Earth directly and has often more extreme counterparts in other planet-hosting star systems.
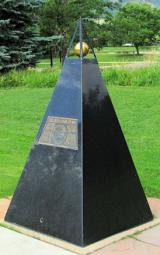
His contributions to the campus include the Scale Model Solar System, an exhibit showing the relative distances and sizes of the planets in our solar system that commemorates the astronauts killed in the space shuttle Challenger explosion in 1986.
1. How did you come to be at CU?
I came to Boulder in the fall of 1971 to enter the graduate program in CU’s physics department after finishing at Harvard College and spending a summer internship at Kitt Peak Observatory in Tucson.
I hadn’t been to Boulder before, and my first view of Boulder Valley from the overlook on the turnpike was unforgettable. A perk of being a grad student in physics was a key to the Reading Room at the top of Duane Tower, which has a commanding view of Folsom Field. I had the privilege of watching, from that lofty perch, CU’s football team rise to No. 3 in the nation that fall, bested only by arch rivals No. 1 Nebraska and No. 2 Oklahoma. (Remember the “wishbone formation”?) It was hard to believe that the top three teams in the country all were from the same conference, the then (not-so-big) Big Eight.
I left CU with a Ph.D. in astrophysics in 1975 but returned a few years later after a stint as a postdoc at Harvard. The lure of the rapidly growing space astronomy program at CU, and perhaps a touch of Chief Niwot’s curse, brought me back. I worked in JILA for a couple of years with my Ph.D. adviser, Jeffrey Linsky, on an early UV space observatory called the International Ultraviolet Explorer. Then, in 1980, I moved over to the Laboratory for Atmospheric and Space Physics (LASP) into a solar UV instrumentation program. In 1985, I and several astronomy colleagues from LASP formed CASA, which is dedicated to studying deep space phenomena beyond our solar system.
2. Some of your research deals with measuring ultraviolet emissions of stars. How are these emissions “seen” and what stars are you studying?
The ultraviolet is beyond the bluest colors that human eyes can see, and, in fact, mostly is blocked by ozone absorption in our Earth’s atmosphere (otherwise, think very bad sunburns). Astronomers must send their instruments beyond the atmosphere into space to record the ultraviolet radiation of stars and other celestial objects.
An important type of instrument is the spectrograph, which works something like a prism to spread out the UV into – to our eyes – an invisible rainbow, which then is captured by special cameras sensitive to that light. The allure of the UV is that it traces high temperatures in cosmic environments. Such hot conditions (five to 100 times the sun’s already toasty 6,000 Kelvin surface temperature) occur naturally on so-called “hot stars,” which are much more massive, luminous, and warmer than the sun and surprisingly also on the sun itself. The sun is a “cool star,” which nonetheless has high-altitude atmospheric layers (something like the Earth’s ionosphere) that can be strongly disturbed by sunspots, vast magnetic “storms” on the solar surface. This magnetic activity creates very hot pockets of gas in the sun’s corona and inspires a variety of explosive phenomena that reach far out into the solar system, creating “space weather.”
These effects, in extreme cases, can be dangerous to our high-technology civilization on Earth. Consequently, the Space Weather Prediction Center (SWPC, pronounced “SwipSee”) at the National Oceanic and Atmospheric Administration (NOAA) in Boulder, keeps a sharp lookout for such events, and issues warnings when trouble is on the way.
Ultraviolet studies of the sun and other stars are one piece of the larger puzzle to understand stellar magnetic activity, partly for the practical reason to improve space weather predictions, and partly to establish the basic physical underpinnings of the phenomena, which are widespread in the cosmos.
A big advantage in the exploration of stellar ultraviolet properties is to examine nearby stars. That is because the closer stars are brighter, while more distant stars are dimmer, so the nearest stars provide the easiest targets. The two most popular UV instruments today both are on Hubble Space Telescope (HST), and the newest one, installed in 2009, was designed at CASA. It’s called Cosmic Origins Spectrograph (COS for short) and was built in Boulder at Ball Aerospace under the direction of principal investigator Jim Green and his CASA team.
COS was designed to achieve the best possible sensitivity, and, in fact, is so sensitive that the nearest stars are too bright for it. The other HST instrument, Space Telescope Imaging Spectrograph (STIS), also built in Boulder, installed in 1997 and refurbished in 2009, is just right for the nearest stars. The closest of the “nearby” stars is the famous Alpha Centauri, only four light years away. Only, in this case, is relative: With our most advanced propulsion systems, a space probe from Earth would take more than 10,000 years to make the trip. The folks in the sci-fi film “Avatar” made the crossing in only six years. (I would love to know that technology.)
Alpha Centauri is a hierarchical-triple system: two heavier stars orbiting each other relatively close together (about 10 times the separation between the sun and Earth), and a lighter companion revolving around the central pair at a great distance (about 10,000 times the Sun-Earth separation). The two bigger stars, A and B, are very similar to our sun. The smaller of the three, C or Proxima, is a diminutive red dwarf, which is not much bigger in size (although much heavier) than Jupiter, the largest planet in our solar system. Ten Proximas could fit across the face of the Sun. In its million-year orbit around AB, Proxima just happens to be on the sun-ward side right now, so has the (somewhat temporary) honor of the nearest star.
Recently, Proxima was reported to host an Earth-size exoplanet in the habitable zone, where liquid water could exist on a planetary surface, which is considered an important pre-condition for life. X-ray studies of Alpha Cen AB by the Chandra Observatory show that these sun-like stars have ups and downs in their high-energy coronal activity similar to the sun’s 11-year sunspot cycle, and that the radiation environments (X-rays and UV) of both stars are not too different from that of the sun (which is not awful, else life would not have thrived on our Earth for eons).
Alpha Cen AB are not yet known to host exoplanets, but if such companions ultimately are found in the habitable zones of either star, there is no high-energy obstacle to the development of life. It’s a different story for Proxima, however, which is categorized as an X-ray flare star, not unusual for these red dwarfs. The bursty, harsh radiation environment in Proxima’s habitable zone, which is very close to the star because red dwarfs produce much less heat at a given planetary distance than the sun, is simply terrible: Potential life forms on Proxima’s planet would be well-advised to grow thick lead shells or live entirely underground.
Curiously, the age of Alpha Centauri probably is about a billion years older than the sun, so it is possible that advanced civilizations might have sprung up there, and fallen, long before even dinosaurs made their appearance on our own planet. One thing is clear: Alpha Centauri will be the first stop by interstellar explorers from Earth, simply because it is so much closer than the next nearest candidates, there are three different stars to visit, and at least one (Proxima) has a potentially habitable planet (putting aside the horrible space weather forecast).
In recent years, I and an international team have carried out major programs with HST’s STIS spectrograph to collect high-quality UV spectra of nearby, bright examples of both hot and cool stars (including Alpha Cen AB) to serve as fundamental references for future observational and theoretical research. This effort is called the Advanced Spectral Library (ASTRAL).
3. How has the study of stars changed over the years and how has this advanced yours and others’ work?
Almost all areas of astronomy, especially in space, are driven by advancements in technology. These range from new camera designs to power more sensitive instruments to Moore’s law that enables, for example, ever more sophisticated computer simulations of the growth of cosmic structure in the early universe.
The first orbiting UV observatories in the late 1970s were smaller than a ski condo refrigerator and could capture only the nearest and brightest stars, and then not particularly well. (But we astronomers were delighted to work with that data then because there was nothing like it before.)
Nowadays, we use state-of-the-art instruments (relatively) recently installed into school-bus-size Hubble. (Although 30 years old, the crucial main telescope mirror still is the best ever made.) With the Boulder-bred COS spectrometer on board, we can reach down to the faintest cataloged cool stars.
Technology has enormously expanded astronomical discovery space, and computing breakthroughs are allowing us to corral this big-data bonanza. Advanced numerical simulations are providing crucial insights into how stars change over time, from their birth in swirling clouds of dust and gas, to slow growth during the long period of internal hydrogen fusion “burning,” to the rapid evolution at the terminal stages when the hydrogen fuel is exhausted. At this endpoint, a sun-like star bloats up into a red supergiant, extending out to the equivalent of Mars’ orbit, then explosively sheds its outer layers, while the insides retreat into a super-condensed hot white dwarf star.
As in many areas of science, technology makes the future of astronomy bright.
4. You note a “fundamental disagreement between helioseismology and surface spectroscopic measurements” on your web page. What is the disagreement about and what side are you on?
There is a long-running controversy in the solar community involving how much oxygen is in the sun – the so-called solar oxygen abundance crisis. Oxygen is important because it is the third most common element in the sun (albeit only at 0.1 percent concentration), after hydrogen (90 percent) and helium (10 percent).
Two independent sides are involved in the debate. One group interprets spectroscopic measurements of the solar atmosphere using sophisticated numerical simulations, something like the National Center for Atmospheric Research’s (NCAR) Global Circulation models applied to the Earth’s weather and climate. The other group images subtle sound-wave patterns on the solar surface and analyzes them in terms of internal properties of the sun, using techniques analogous to those that geophysicists deploy to probe the inside of the Earth with seismic waves.
The spectroscopists directly observe atmospheric spectral signatures sensitive to elemental oxygen, whereas the helio-seismologists have a less-direct way to measure oxygen, but the nuts and bolts of their technique is much simpler. The disagreement is about a factor of 2, with the spectroscopists on the low side. Normally, a mere factor of 2 disagreement in astronomy, where conflicts involving factors of 10 are common, would be heralded as a great achievement. But this is the sun, and the chemical compositions of all the other stars are pegged relative to it. So, this was a key issue.
About 10 years ago, I performed an independent evaluation of the spectroscopic analysis, and, unexpectedly, came up with a result much higher than the spectroscopic oxygen abundance, closer to the seismic value. In the process, I identified several aspects of the spectroscopic approach that were subject to perhaps questionable, and difficult-to-quantify, assumptions. Even though I am a card-carrying spectroscopist by trade, my conclusion was that the atmospheric spectroscopy was inherently too messy to be blindly trusted, and that the more straightforward internal seismology likely was more reliable. Thus, I have defected to the high-O side (“O” for oxygen). I currently have a bet – a bottle of fine Scotch – with one of my low-O colleagues on the eventual outcome of the debate.
5. What is a favorite item you have in your office that holds special meaning for you?
Propped on a shelf in my Duane office is a trapezoidal bronze plaque that was a prototype for a 1987 project called the Scale Model Solar System (SMSS) at CU. My grad student at the time, Jeff Bennett, and I had taken on the project to commemorate aerospace engineering alum Ellison Onizuka and the other astronauts who perished in the shuttle Challenger disaster the year before.
The SMSS was intended to depict the relative distances and sizes of the sun and the planets on the same scale, 1:10 billion. (For you math whizzes, that’s 1 to 10 to the 10th power.) On this scale, the sun is the size of a grapefruit; Earth, the head of a ballpoint pen about 15 yards away; Jupiter, a marble about a football field away; Alpha Centauri A, another grapefruit all the way down in Panama.
The exhibit consists of an array of black granite pedestals, one for each planet (counting Pluto, which had not yet been demoted to dwarf planet stature at that time) on the path extending from Fiske Planetarium about a half-mile north to Colorado Avenue next to the Engineering Building.
Jeff and I were looking for ways to display information for each planet, and our first idea was to use bronze plaques, which have a lot of advantages for an outdoor display in Colorado’s notoriously harsh weather. Jeff enlisted the aid of a graduate student in the Fine Arts Department to cast a test plate for the central pyramid of the display (topped by the model sun: a polished, yellow-anodized aluminum sphere, courtesy of LASP). We paid the student $4,000 for the summer, from a construction fund graciously provided by then-CU President Gordon Gee. The final test plaque, after three month’s work, was artistically stunning, but difficult to read and the cost was too high. In other words, an unmitigated disaster.
Jeff and I turned to a different approach: fiberglass panels with text and pictures under three-quarter-inch thick glass plates, with the scale-model planets cut as tiny hemispheres into the underside of the plates. The result was visually beautiful and super-informative, but unfortunately, within a few months, became water-logged thanks to an unforeseen bad confluence of solar heating and Facilities Management lawn sprinklers.
A few years later, when Jeff had left Boulder for NASA Headquarters in Washington to oversee educational programs including a new SMSS project for the Washington Mall, I managed to secure a grant to replace the failed glass plates with bronze plaques, this time professionally casted. A talented undergraduate student, Jodi Schoemer, was responsible for most of the design work on the new plaques, which have graced the granite pedestals for the nearly three decades since. Jodi currently is director of Experiences and Partnerships at Denver’s Museum of Nature and Science.
Meanwhile, Jeff Bennett has become a noted astronomy textbook and popular science writer; his undergraduate texts continue to be used in my department (APS). I still have the original flawed SMSS bronze plaque to remind me, as I’m sure Thomas Edison would agree: Experimentation does not always succeed and failures at times can be expensive, but if you keep at it, something eventually will work.
Incidentally, there is an effort afoot, led by current APS grad student Jimmy Negus, to replace the current SMSS with an updated, state-of-the-art display. This would be an awesome enhancement to the educational experience of CU undergrads and the many visitors to our campus as well.